Ice and firn heterogeneity within Larsen C Ice Shelf from borehole optical televiewing
Ice shelves fringe much of Antarctica and are particularly sensitive indicators of environmental changes owing to their low elevation, low latitude, and exposure to both oceanic and atmospheric forcings. Ice shelves form when glaciers and ice streams flowing across the grounding line begin to float and spread laterally to fill an embayment. Once established by this core of continental ice, they are able to gain and lose mass at their upper and lower interfaces, as well as calving at the marine margin. Although, ice shelves are floating and therefore experience negligible basal shear stress, lateral stresses, and intermittent pinning to the seabed result in an ability to buttress grounded ice upstream. Through this, ice shelves modulate the contribution of the Antarctic Ice Sheet to eustatic sea level rise. Where ice shelves have thinned or collapsed, inflowing glaciers have accelerated and their discharge has increased accordingly [e.g., Paolo et al., 2015; De Rydt et al., 2015; Scambos et al., 2004].
A critical characteristic of ice shelves is their predisposition to lose mass episodically once their geometry becomes unstable [Kulessa et al., 2014]. The end-member of this behavior is a near-total disintegration of ice shelves, exemplified by the collapse of Larsen B Ice Shelf in early 2002 [Scambos et al., 2003]. The disintegration of Antarctic Peninsula ice shelves has progressed poleward for the last ~30 years, commensurate with climate warming [Cook and Vaughan, 2010], and the mean annual isotherm of −9°C has been proposed as a practical limit of viability for ice shelves [Morris and Vaughan, 2003]. The largest remaining Antarctic Peninsula ice shelf, Larsen C Ice Shelf (LCIS), is intersected by this isotherm and may be currently experiencing surface melting conditions similar to those that preceded the collapse of its neighbors: Larsen A in 1995 and Larsen B in 2002. The surface of LCIS has lowered during the satellite era. Recent work has indicated that approximately half of this lowering signal is caused by the loss of air from the ice shelf firn [Holland et al., 2015]. This air loss may be related to decreased surface accumulation or enhanced melting or firn compaction associated with Antarctic Peninsula warming [Turner et al., 2016]. Proposed mechanisms of ice shelf disintegration commonly invoke intense surface melting and ponding leading to flexure and (hydro)fracture [Banwell et al., 2013; Scambos et al., 2009]. In order for melt ponds to form, snowpack pore space must first be first filled [Kuipers Munneke et al., 2014] or a substantial layer of impermeable refrozen (infiltration) ice must exist [e.g., Machguth et al., 2016]. Distributed estimates of ice shelf thickness, a fundamental input into glaciological models, are typically derived through satellite altimetry by way of an assumption of hydrostatic equilibrium, requiring a correction to account for the proportion of the ice column not made up of pure ice. The term “firn air” is used to conceptualize both the mean density of the full ice column as the equivalent reduction in ice thickness if it were completely composed of bubble-free glacial ice and the pore space available for meltwater storage in the near surface. It is important to note that these definitions are not identical as material above the pore closure density and below the density of bubble-free glacial ice will contribute to firn air according to the former definition but not the latter.
....
Results of this study indicate that föhn winds and seasonal melting are a major influence on the physical structure of LCIS, which may have implications for ice shelf evolution, modeling, and stability.
Related Content
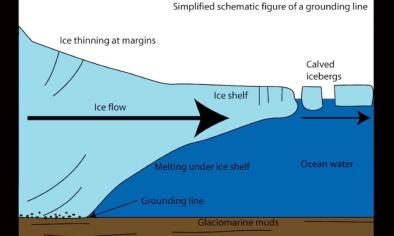
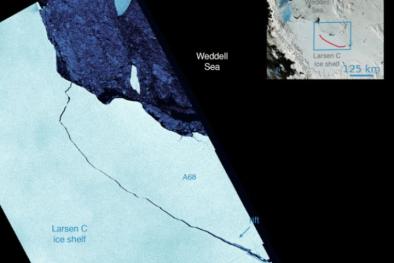
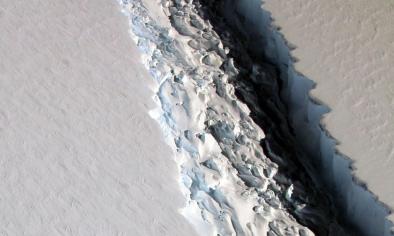
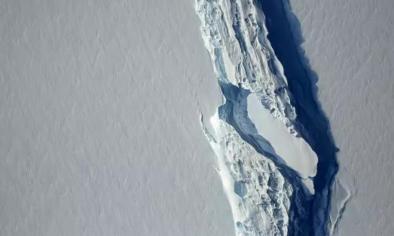